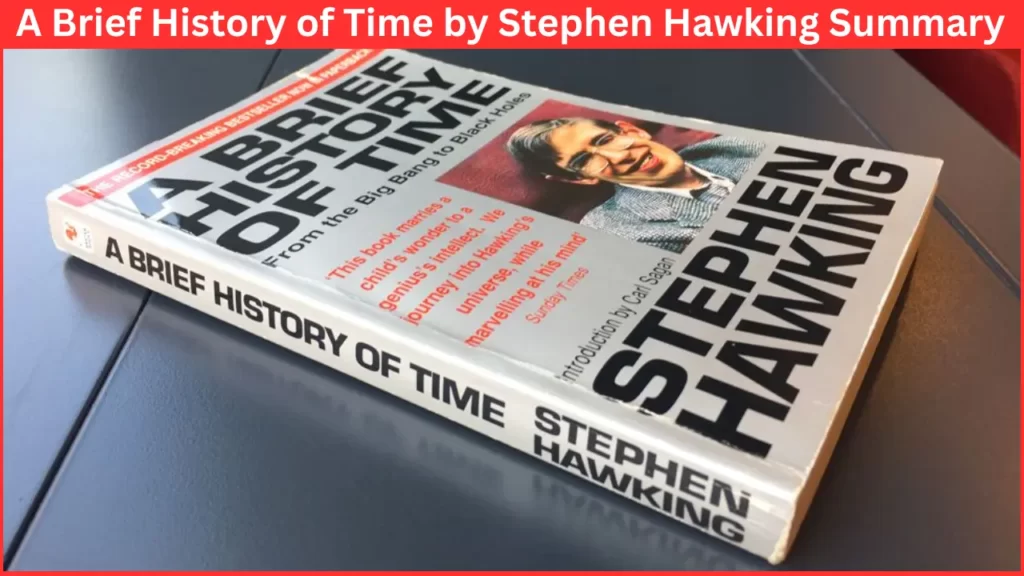
A well-known scientist, possibly Bertrand Russell, once gave a lecture on astronomy to the public. He talked about how the earth goes around the sun and how the sun goes around the center of our galaxy, a huge collection of stars. A small elderly woman in the back of the room stood up at the lecture’s conclusion and said, Your statements to us are false.
In reality, the world is just a flat plate supported by a huge tortoise.” Before responding, the scientist gave a superior grin and asked, “What is the tortoise standing on?” The elderly lady exclaimed, “You’re very clever, young man, very clever.” However, turtles rule the bottom! The image of our universe as an infinite tower of tortoises would be absurd to the majority of people, but why do we think we know better? How do we know what we know about the universe? Where did the universe come from, and where is it going? If there was a beginning to the universe, what happened before that? What is the idea of time? Is it ever going to end? Could we at any point travel once more into the past? Some of these enduring inquiries may now have answers thanks to recent physics innovations that were made possible in part by amazing new technologies.
Sometime these responses might appear to be as clear to us as the earth circling the sun – or maybe as ludicrous as a pinnacle of turtles. Just time (anything that might be) will tell. As some time in the past as 340 BC the Greek logician Aristotle, in his book On the Sky, had the option to advance two great contentions for accepting that the earth was a round circle as opposed to a Cap plate. He first realized that the earth passing between the sun and the moon caused lunar eclipses. Only if the earth were spherical would the earth’s shadow on the moon always be round.
Unless the eclipse always occurred when the sun was directly under the center of the disk, the shadow would have been elongated and elliptical if the earth had been a flat disk. Second, the Greeks were aware from their travels that when viewed from the south, the North Star appeared lower in the sky than when viewed from more northerly regions. The North Star appears to be directly above an observer at the North Pole because it is above the pole; however, from the equator, it appears to be just above the horizon. Aristotle even estimated the circumference of the earth to be 400,000 stadia based on the difference in apparent North Star position between Egypt and Greece.
A stadium’s exact length is unknown, but it may have been around 200 yards, making Aristotle’s estimate approximately twice the current consensus. The Greeks even had a third argument: the earth must be round because there is no other explanation for how a ship’s sails appear first and then its hull. Aristotle believed that the earth was stationary and that the sun, moon, planets, and stars moved around the earth in circular orbits. He accepted this since he felt, for mysterious reasons, that the earth was the focal point of the universe, and that roundabout movement was the absolute best. In the second century AD, Ptolemy developed this concept into a comprehensive cosmological model.
The moon, the sun, the stars, and the five planets that were known at the time—Mercury, Venus, Mars, Jupiter, and Saturn—were carried by eight spheres around the earth at its center. To explain their rather complicated observed paths in the sky, the planets themselves moved in smaller circles attached to their spheres. The so-called fixed stars, which rotate together across the sky but always remain in the same relative positions, were carried by the outermost sphere. It was never made very clear what lay beyond the last sphere, but it was certainly not a part of the universe that humans could observe. Ptolemy’s model offered a method for predicting the positions of celestial bodies in the sky with some degree of accuracy.
But Ptolemy had to assume that the moon moved in a way that sometimes brought it twice as close to the earth as other times in order to correctly predict these positions. As a result, the moon ought to appear twice as big at different times! Despite the fact that Ptolemy was aware of this flaw, his model was generally, if not always, accepted. It had the great advantage of leaving a lot of space outside the sphere of fixed stars for heaven and hell, so it was adopted by the Christian church as the universe depiction that was in line with Scripture.
According to Newton’s theory of gravity, the stars should be attracted to one another, so it appeared that they could not remain motionless. Wouldn’t they all eventually come together? Newton argued that this would occur if there were only a finite number of stars spread out over a finite area of space in a 1691 letter to Richard Bentley, another prominent thinker of the time. However, he reasoned that this would not occur if, on the other hand, there were an infinite number of stars evenly distributed across an infinite space because there would be no central point from which they could fall.
One illustration of the problems that can arise when discussing infinity is this argument. Because each point has an infinite number of stars on either side, it can be considered the center of an infinite universe. It wasn’t until much later that it was realized that the correct method is to first think about the limited situation in which all of the stars collide, and then to ask how things change if more stars are added that are roughly evenly distributed outside of this region. The stars would fall in just as quickly because, in accordance with Newton’s law, the additional stars would, on average, have no effect at all on the original ones.
We are free to add as many stars as we want, but they will always collapse. We now know that there is no such thing as an infinite static universe model in which gravity is always appealing. The fact that no one had ever proposed that the universe was expanding or contracting is an interesting reflection on the general climate of thought prior to the twentieth century. It was generally agreed that either the universe had existed in an unchanging state for all time or that it had been created at a limited time in the past, similar to how it is now.
This may have been in part due to people’s propensity to believe in eternal truths and the consolation they derive from the idea that the universe is eternal and unchanging regardless of whether they live or die. The German philosopher Heinrich Olbers, who wrote about this theory in 1823, is typically credited with another objection to it. In fact, a number of Newton’s contemporaries had raised the issue, and the Olbers article was not even the first to present plausible counterarguments. It was, in any case, the first to be generally noted.
The problem is that nearly every line of sight would end on a star’s surface in an infinite, static universe. As a result, even at night, one would expect the sky to be as bright as the sun. Olbers’ counter-contention was that the light from far off stars would be darkened by retention by mediating matter. However, in that case, the matter in between would eventually heat up to the point where it would shine as brightly as the stars. The only way to avoid coming to the conclusion that the entire night sky should be as bright as the sun’s surface is to assume that the stars did not shine continuously but instead turned on at a specific point in time. If that were the case, either the light from distant stars or the absorbing matter might not have yet heated up.
This brings us to the issue of what might have triggered the stars’ initial activation. You need to know what a scientific theory is in order to discuss topics like the nature of the universe and whether it has a beginning or end. I’ll go with the unassuming viewpoint that a theory is merely a model of the universe, or a small portion of it, and a set of rules that connect quantities in the model to our observations. It exists just to us and has no other reality (anything that could mean). A good theory is one that meets two requirements.
On the basis of a model with only a few arbitrary elements, it must accurately describe a large class of observations and make specific predictions about the outcomes of future observations. Aristotle, for instance, subscribed to Empedocles’ theory that there were four elements in nature: water, air, fire, and earth. Despite being straightforward, this did not provide any definitive predictions. Newton’s theory of gravity, on the other hand, was based on a model that was even simpler: two bodies attracted to each other with a force that was inversely proportional to the square of the distance between them and proportional to their mass. However it predicts the movements of the sun, the moon, and the planets to a serious level of precision.
Any actual hypothesis is generally temporary, as in it is just a theory: It will never be proven. You can never be certain that the subsequent result will not contradict the theory, regardless of how many times the results of experiments agree with the theory. On the other hand, even a single observation that goes against the theory’s predictions can be used to disprove a theory. A good theory, as the scientist and philosopher Karl Popper has emphasized, is one that makes a number of predictions that could theoretically be disproved or falsified by observation. Each time new trials are seen to concur with the expectations the hypothesis makes due, and our trust in it is expanded; in any case, if at any point a novel perception is found to deviate, we need to leave or alter the hypothesis.
Today researchers portray the universe concerning two fundamental fractional hypotheses – the overall hypothesis of relativity what’s more, quantum mechanics. They are the greatest intellectual accomplishments of this century’s first half. The force of gravity and the large-scale structure of the universe, that is, the structure on scales as small as a few miles to as large as a million million million million (1 with twenty-four zeros after it) miles, the size of the observable universe, are both described by the general theory of relativity. Quantum mechanics, then again, manages peculiarities for minuscule scopes, like a millionth of a millionth of an inch. Sadly, however, it is established that these two theories are incompatible; they cannot both be correct.
The search for a new theory that will incorporate them both, a quantum theory of gravity, is one of the most important current endeavors in physics and the central theme of this book. Although we do not yet possess such a theory and may still be a long way from having one, we do already know many of the properties that it must possess. Furthermore, we will see, in later parts, that we definitely know a decent lot about the predications a quantum hypothesis of gravity must make. Now, if you think that the universe is controlled by specific laws and is not random, you will eventually have to combine the different theories into a single, comprehensive theory that will explain everything in the universe.
The search for such a comprehensive unified theory, on the other hand, is fundamentally contradictory. The aforementioned concepts about scientific theories assume that we are rational beings who are free to observe the universe however we please and to make logical deductions from what we observe. It is reasonable to assume that under such a plan, we might get closer and closer to the universe’s rules. However, if a complete unified theory existed, it would probably also dictate our actions.
Thus the actual hypothesis would decide the result of our quest for it! And why should it be the one to decide whether or not the evidence supports our conclusions? Is it not possible that it could also determine that we arrive at the wrong conclusion? Or nothing at all? Based on Darwin’s theory of natural selection, I can only offer one solution to this issue. The idea is that different people’s genetic makeup and upbringing will vary in any population of organisms that reproduce themselves. Because of these differences, some people will be better than others at understanding the world around them and acting accordingly. Because these people will be more likely to live and reproduce, their way of thinking and acting will take over.
It has surely been valid in the past that what we call knowledge and logical disclosure have conveyed a endurance advantage. It isn’t the case clear that this is as yet the situation: our logical disclosures might well obliterate all of us, furthermore, regardless of whether they, a total brought together hypothesis may not have a lot of effect on our possibilities of endurance. However, given that the universe has evolved in a consistent manner, we can anticipate that the reasoning skills that natural selection has given us will also be valid in our search for a comprehensive unified theory and will not lead us astray. Newton’s laws of motion were founded on Galileo’s measurements.
In Galileo’s experiments, the same force—a body’s weight—always acted on it as it rolled down the slope, causing it to always accelerate. This demonstrated that, contrary to what was previously thought, the actual effect of a force is always to alter a body’s speed rather than simply to initiate movement. It also meant that a body will continue to move in a straight line at the same speed whenever there is no force acting on it. This concept was first expressed explicitly in 1687 edition of Newton’s Principia Mathematica, which is also known as Newton’s first law. Newton’s second law explains what happens to a body when a force does act on it. According to this, the body will accelerate, also known as change its speed, at a rate that is inversely proportional to the force.
For instance, the speed increase is two times as perfect on the off chance that the power is two times as perfect.) The acceleration also decreases with increasing body mass (or quantity of matter). A body with twice as much mass will accelerate half as much with the same force.) A car serves as an illustrative illustration: Acceleration is proportional to engine power, but acceleration is proportional to vehicle weight for the same engine. Newton discovered a law to describe the force of gravity in addition to his laws of motion.
This law states that every body attracts every other body with a force proportional to its mass. As a result, if one of the bodies, say body A, had twice the mass, the force between them would be twice as strong. This is to be expected because the new body A could be thought of as being made up of two bodies with the same mass. Body B would be attracted by each using the initial force. As a result, the combined force between A and B would be twice as strong as it was before. The force would also be six times stronger if, for instance, one of the bodies had twice as much mass and the other had three times as much mass.
One can presently see the reason why all bodies fall at a similar rate: A body with twice as much mass as a body with twice as much weight will have twice as much force pulling it down. The acceleration will always be the same because, in accordance with Newton’s second law, these two effects will precisely cancel one another. We also know from Newton’s law of gravity that the force is smaller the further apart the bodies are. According to Newton’s law of gravity, a star’s gravitational attraction is exactly one quarter that of a star of the same mass at half the distance.
The orbits of the earth, the moon, and the planets are accurately predicted by this law. Planetary orbits would not be elliptical if the law of gravity dictated that a star’s gravitational attraction decreased or increased more rapidly with distance. Instead, they would either spiral toward the sun or escape from it. It was impossible to determine whether two events that occurred at different times took place in the same space because there was no absolute rest standard. Let’s say, for instance, that our train-bound Ping-Pong ball bounces in a straight line and hits the table twice, one second apart.
Due to the fact that the train would have traveled that far down the track between the two bounces, it would appear to someone on the track that they occurred approximately forty meters apart. The nonexistence of outright rest accordingly intended that one couldn’t give an occasion an outright situation in space, as Aristotle had accepted. There would be no reason to favor one person’s position over the other because the positions of events and distances between them would differ for those on the train and those on the track. Newton was exceptionally stressed by this absence of outright position, or outright space, as it was called, on the grounds that it did not accord with his concept of an outright God.
In fact, despite the fact that his laws implied it, he refused to accept absolute spacelessness. Many people strongly criticized him for this irrational belief, most notably Bishop Berkeley, a philosopher who held the belief that all physical things, space, and time are illusions. The well-known Dr. Johnson yelled, “I refute it thus!” when he heard Berkeley’s opinion. and injured his toe by stepping on a large stone. Newton and Aristotle both held the view of absolute time. That is, they believed that anyone could accurately measure the time that passed between two events and that, as long as they used a reliable clock, this time would remain the same for everyone. Space and time were completely distinct entities.
The view that most people would consider to be common sense is this. However, we have had to alter our perceptions of time and space. When dealing with things like apples or planets that travel relatively slowly, our seemingly common sense ideas work well, but they don’t work at all for things moving at or close to the speed of light. The central propose of the hypothesis of relativity, as it was called, was that the laws of science ought to be the equivalent for all unreservedly moving onlookers, regardless of what their speed.
This was valid for Newton’s laws of movement, yet presently the thought was stretched out to incorporate Maxwell’s hypothesis and the speed of light: No matter how quickly they are moving, all observers ought to measure the same speed of light. This straightforward thought has some momentous results. The law that nothing can travel faster than the speed of light and the equivalence of mass and energy, summed up in Einstein’s famous equation E=mc2 (where E is energy, m is mass, and c is the speed of light), are perhaps the most well-known.
The motion of an object will add energy to the object’s mass due to the equivalence of energy and mass. At the end of the day, it will make it harder to speed up. Objects traveling at speeds close to the speed of light are the only real beneficiaries of this effect. An object’s mass, for instance, is only 0.5% higher than normal at 10% of the speed of light, whereas it would be more than twice as high at 90% of the speed of light. Because an object’s mass rises at a faster rate as it approaches the speed of light, it needs more and more energy to accelerate further. It can as a matter of fact never come to the speed of light, on the grounds that by then its mass would have become limitless, and by the comparability of mass and energy, it would have taken a limitless measure of energy to get it there. Because of this, the laws of relativity prevent any normal object from ever moving faster than the speed of light. At the speed of light, only light or other waves without mass can move.